Protein catabolism
Protein catabolism is the breakdown of proteins into amino acids and simple derivative compounds, for transport into the cell through the plasma membrane and ultimately for the polymerization into new proteins via the use of ribonucleic acids (RNA) and ribosomes. Protein catabolism, which is the breakdown of macromolecules, is essentially a digestion process.
Protein catabolism is most commonly carried out by non-specific endo- and exo-proteases. However, specific proteases are used for cleaving of proteins for regulatory and protein trafficking purposes. One example is the subclass of proteolytic enzymes called oligopeptidase.
The amino acids produced by catabolism may be directly recycled to form new proteins, converted into different amino acids, or can undergo amino acid catabolism to be converted to other compounds via the Krebs cycle.[1]
Purpose
The primary reason for protein catabolism is so organisms can convert proteins into a form of energy that they can use or store. To reuse their proteins, bacteria or soil microorganisms break down their proteins through protein catabolism into their individual amino acids and are used to form bacterial proteins or oxidized for energy. To convert to energy, once the proteins are broken down, they are typically deaminated (removal of an amino group) so that they can be processed into the Krebs/Citric Acid (TCA) Cycle. By proceeding into the Citric Acid Cycle, the original proteins will be converted into usable energy for the organism.[1]
There are also other processes to convert amino acids into usable molecules to enter the TCA cycle, such as transamination (transfer of amino group), decarboxylation (removal of carboxyl group), and dehydrogenation (removal of hydrogen).[1]
The proteins are digested in the intestines to produce the amino acids. The proteins are continuously being broken down and reformed, depending on the current needs that the body has. Proteins have different half-lives:[2] some have an incredibly short half-life while others have longer ones. Those with short life-lives are primarily used in metabolic pathways or processes because they help the cell adjust continuously and quickly to the changes that occur due to these processes.[3][4]
Protein degradation
The degradation of proteins occurs within the cells, as the amino acids have to pass through certain membranes before being able to be used for different processes. This first step to protein catabolism is breaking the protein down into amino acids by cleaving their peptide bonds, also known as proteolysis. The peptide bonds are broken up by the proteasome, which is able to hydrolyze the peptide bonds by using ATP energy. This process is further helped through the use of enzymes called proteases. The proteases help cleave off the remaining peptide residues to produce individual amino acids, ready to be converted into usable molecules for either glycolysis or the TCA cycle, to produce energy for the organisms, or to be used to create new proteins.[3]
In certain organisms, such as bacteria, the proteins must undergo proteolysis before the amino acids can be re-polymerized into new proteins, because the original proteins cannot pass through the bacterial plasma membrane, as they are too large. After the proteins are broken down into amino acids through proteolysis, these amino acids will be able to pass through the membranes of bacteria and will once again congregate to form new proteins that the bacteria needs to function.[1]
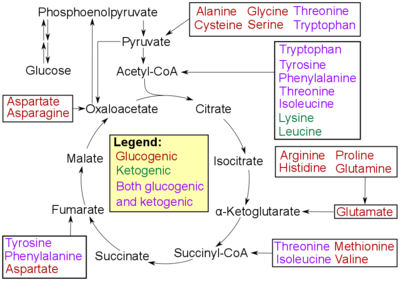
Amino Acid Degradation
Oxidative deamination is the first step to breaking down the amino acids so that they can be converted to sugars. The process begins by removing the amino group of the amino acids. The amino group becomes ammonium as it is lost and later undergoes the urea cycle to become urea, in the liver. It is then released into the blood stream, where it is transferred to the kidneys, which will secrete the urea as urine.[5][6] The remaining portion of the amino acid becomes oxidized, resulting in an alpha-keto acid. The alpha-keto acid will then proceed into the TCA cycle, in order to produce energy. The acid can also enter glycolysis, where it will be eventually converted into pyruvate. The pyruvate is then converted into acetyl-CoA so that it can enter the TCA cycle and convert the original pyruvate molecules into ATP, or usable energy for the organism.[7]
Transamination leads to the same end result as deamination: the remaining acid will undergo either glycolysis or the TCA cycle to produce energy that the organism's body will use for various purposes. This process transfers the amino group instead of losing the amino group to be converted into ammonium. The amino group is transferred to alpha-ketoglutarate, so that it can be converted to glutamate. Then glutamate transfers the amino group to oxaloacetate. This transfer is so that the oxaloacetate can be converted to aspartate or other amino acids. Eventually, this product will also proceed into oxidative deamination to once again produce alpha-ketoglutarate, an alpha-keto acid that will undergo the TCA cycle, and ammonium, which will eventually undergo the urea cycle.[3]
Transaminases are enzymes that help catalyze the reactions that take place in transamination. They help catalyze the reaction at the point when the amino group is transferred from the original amino acid, like glutamate to alpha-ketoglutarate, and hold ono it to transfer it to another alpha-ketoacid.[3]
See also
References
- 1 2 3 4 Bauman, Robert W.; Machunis-Masuoka, Elizabeth; Tizard, Ian R. (2004-01-01). Microbiology. Pearson/Benjamin Cummings. ISBN 9780805376524.
- ↑ Zhou, Pengbo (2004-01-01). Dickson, RobertC.; Mendenhall, MichaelD., eds. Signal Transduction Protocols. Methods in Molecular Biology. Humana Press. pp. 67–77. doi:10.1385/1-59259-816-1:067. ISBN 9781588292452.
- 1 2 3 4 Miles, Bryant (April 9, 2003). "Protein Catabolism" (PDF).
- ↑ Bojkowska, Karolina; Santoni de Sio, Francesca; Barde, Isabelle; Offner, Sandra; Verp, Sonia; Heinis, Christian; Johnsson, Kai; Trono, Didier (2011-06-24). "Measuring In Vivo Protein Half-Life". Chemistry & Biology. 18 (6): 805–815. doi:10.1016/j.chembiol.2011.03.014.
- ↑ "26.9: The Catabolism of Proteins". Chemistry LibreTexts. 2014-06-19. Retrieved 2016-10-25.
- ↑ "Oxidative Deamination". chemistry.elmhurst.edu. Retrieved 2016-10-25.
- ↑ "GLYCOLYSIS AND THE KREBS CYCLE". homepage.smc.edu. Retrieved 2016-11-08.