Secretion of creosote preservative into aquatic ecosystem
.jpg)
There are many concerns about the environmental impact of the secretion of creosote preservative into the aquatic ecosystem. Creosote, a product of the distillation of tar, or thermal decomposition of plant or wood based materials,[1] is used as a chemical preservative in wooden, marine pilings. In a study from the Environmental Protection Agency in 1984, 900 million pounds of wood preservatives were used in both agricultural and industrial productions.[2] This wood preservation is one of the many techniques used to extend the life of the marine piling by making it more durable to weathering and resistant to insects and fungi.[3] Due to its resistance to insects and fungi, the creosote used in marine pilings is labeled as a pesticide.[3] The creosote-soaked wood has a black, oily coating on the outside of the piling and is applied by pressurized treatment.[3] The coating is composed of numerous chemicals which include: phenol, o-cresol, p-cresol, m-cresol, o-ethylphenol, guaiacol, 1,3,4-xylenol, 1,3,5-xylenol, and creosol.[4] These chemicals in the preservative are slowly secreted into the environment from the pilings through destruction or breaking of the wood.[4] The escape of these chemicals creates a hazard to the environment through reactions with the aquatic sediments.
History
Creosote was first used in the 18th century as a preservative on ships.[5] The creosote was wood-tar based, which means that its origin comes from the combustion of wood or plant materials.[5] The preservatives protected the ships on long voyages across the oceans.[5] This preservative allowed for the ships to resist weathering and natural forces that occurred during the travel.[5] In the 1830's, German scientists began experimenting with wood-tar based creosote for medicinal purpose.[6] These scientists used the wood-tar with various forms of herbal remedies for medical conditions relating to everything from lung to external injuries.[6] After the durability of the creosote was seen on these ships, and its versatile medical remedies were noted, it began being industrialized for more commercial uses.[5] Issues with the industrialization arouse when creosote could not be administered into the wood, only to its outer surface.[5] In 1906, the use of pressurization was introduced in order to administer the creosote deeper into the wood through small pores.[5] These pressurization techniques were used for telephone poles, building structures, and marine pilings.[5] Creosote became an official wood preservative in 1948.[3] The American Wood Protection Association placed standards on the creosote preservatives and the pressurization techniques that regulated the amount of chemicals used in the process.[5] In 2008, the Environmental Protection Agency banned the use of three compounds, including creosote, citing that occupational and ecological exposures have been linked to environmental hazards.[7]
Release of Creosote

Even though creosote is pressurized into the wood, the release of the chemical can be seen from many different events. During the lifetime of the marine piling, weathering occurs from tides and water flow which slowly opens the oily outer coating and exposes the smaller internal pores to more water flow.[8] Frequent weathering occurs daily, but more severe weather, such as hurricanes, can cause damage or losening of the wooden pilings.[8] Many pilings are either broken into pieces from debris, or are completely washed away during these storms. When the pilings are washed away, they come to settle on the bottom of the body of water where they reside, and then they secrete chemicals into the water slowly over a long period of time. This long term secretion is not normally noticed because the piling is submerged beneath the surface hidden from sight. The creosote is mostly insoluble in water, but the lower molecular weight compounds will become soluble the longer the broken wood is exposed to the water.[9] In this case, some of the chemicals now become water soluble and further leach into the aquatic sediment while the rest of the insoluble chemicals remain together in a tar-like substance.[9] Another source of damage comes from insects such as Shipworms and Limnoria.[10] Though creosote is used as a pesticide preservative, studies have shown that Limnoria is resistant to wood preservative pesticides and can cause small holes in the wood which creosote can then be secreted from.[10]
Chemical reactions with sediment and organisms
Once the soluble compounds from the creosote preservative leach into the water, the compounds begin reacting with the external environment or are consumed by organisms. The reactions vary depending on the concentration of each compound that is released from the creosote, but major reactions are outlined below:
Alkylation
Alkylation occurs when a molecule replaces a hydrogen atom with an alkyl group that generally comes from an organic molecule.[11] Alkyl groups that are found naturally occurring in the environment are organometallic compounds.[12] Organometallic compounds generally contain a methyl, ethyl, or butyl derivative which is the alkyl group that replaces the hydrogen.[12] Other organic compounds, such as methanol, can provide alkyl groups for alkylation.[13] Methanol is found naturally in the environment in small concentrations, and has been linked to the release from biological decomposition of waste and even a byproduct of vegetation.[14] The following reactions are alkylations of soluble compounds found in creosote preservatives with methanol.
m-Cresol
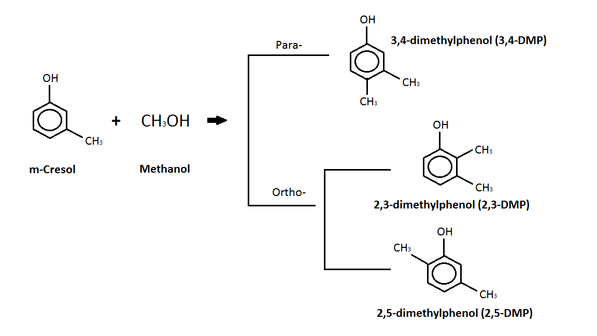
The diagram above depicts a reaction between m-cresol and methanol where a c-alkylation product is produced.[13] The c-alkylation reaction means that instead of replacing the hydrogen atom on the -OH group, the methyl group (from the methanol) replaces the hydrogen on a carbon in the benzene ring.[13] The products of this c-alkylation can be in either a para- or ortho- orientation on the molecule, as seen in the diagram, and water, which is not shown.[13] Isomers of the dimethylphenol (DMP) compound are the products of the para- and ortho-c-alkylation.[13] Dimethylphenol (DMP) compound is listed as an aquatic hazard by characteristic, and is toxic with long lasting effects.[15]
Phenol
This diagram shows an o-alkylation between phenol and methanol. Unlike the c-alkylation, the o-alkylation replaces the hydrogen atom on the -OH group with the methyl group (from the methanol).[16] The product of the o-alkylation is methoxybenzene, better known as anisole, and water, which is not shown in the diagram.[16] Anisole is listed as an acute hazard to aquatic life with long term effects.[17]
Bioaccumulation
Bioaccumulation is the process by which an organism takes in chemicals through ingestion, exposure, and inhalation.[18] Bioaccumulation is broken down into bioconcentration (uptake of chemicals from just water) and biomagnification (uptake of chemicals from a food source).[18] Certain species of aquatic organisms are affected differently from the chemicals released from creosote preservatives. One of the more studied organisms is a mollusk. Mollusks attach to the wooden, marine pilings and are in direct contact with the creosote preservatives.[19] Many studies have been conducted using Polycyclic aromatic hydrocarbons (PAH), which are low molecular hydrocarbons found in some creosote-based preservatives. In a study conducted from Pensacola, Florida, a group of native mollusks were kept in a controlled environment, and a different group of native mollusks were kept in an environment contaminated with creosote preservatives.[20] The mollusks in the contaminated environment were shown to have a bioaccumulation of up to ten times the concentration of PAH than the control species.[20] The intake of organisms is dependent on whether the compound is in an ionized or an un-ionized form.[21] To determine whether the compound is ionized or un-ionized, the pH of the surrounding environment must be compared to the pKa or acidity constant of the compound.[21] If the pH of the environment is lower than the pKa, then the compound is un-ionized which means that the compound will behave as if it is non-polar.[21] Bioaccumulation for un-ionized compounds comes from partitioning equilibrium between the aqueous phase and the lipids in the organism.[21] If the pH is higher than the pKa, then the compound is considered to be in the ionized form.[21] The un-ionized form is favored because the bioaccumulation is easier for the organism to intake through partitioning equilibrium.[21] The table below shows a list of pKas from compounds found in creosote preservatives and compares them to the average pH of seawater (reported to be 8.1).[22]
Compound | pKa | pH of Seawater | Form (Ionized or Un-Ionized) |
---|---|---|---|
m-cresol | 10.09 | 8.1 | Un-ionized |
o-cresol | 10.29 | Un-ionized | |
p-cresol | 10.30 | Un-ionized | |
o-ethylphenol | 10.20 | Un-ionized | |
guaiacol | 9.98 | Un-ionized | |
phenol | 9.99 | Un-ionized |
Each of the compounds in the table above are found in creosote preservatives, and are all in the favored un-ionized form. In another study, various species of small fish were tested to see how the exposure time to PAH chemicals affected the fish.[23] This study showed that an exposure time of 24–96 hours on various shrimp and fish species affected the growth, reproduction, and survival functions of the organisms for most of the compounds tested.[23]
Biodegradation
Biodegradation is the process by which bacteria or fungi in an environment consumes chemicals found in the sediment.[24] This is a form of waste management in the soil which allows for chemicals to be converted or decomposed by the organisms in sediment.[24] This process take place in either anaerobic or aerobic settings which decomposes the chemicals differently.[24] It can be seen in some studies that biodegradation accounts for the absence of creosote preservatives on the initial surface of the sediment.[20] In a study from Pensacola, Florida, PAHs were not detected on the surface on the aquatic sediment, but the highest concentrations were detected at a depth of 8-13 centimeters.[20] A form a anaerobic biodegradation of m-cresol was seen in a study using sulfate-reducing and nitrate-reducing enriched environments.[25] The reduction of m-cresol in this study was seen in under 144 hours, while additional chemical intermediates were being formed.[25] The chemical intermediates were formed in the presents of bicarbonate. The products included 4-hydroxy-2-methylbenzoic acid and acetate compounds.[25] Although the conditions were enriched with the reducing anaerobic compounds, sulfate and nitrate reducing bacteria are commonly found in the environmentnt. For further information, see sulfate-reducing bacteria. The type of anaerobic bacteria ultimately determines the reduction of the creosote preservative compounds, while each individual compound may only go through reduction under certain conditions.[26] BTEX is a mixture of benzene, toluene, ethylbenzene, and xylene, that was studied in the presence of four different anaerobic-enriched sediments.[26] Though the compound, BTEX, is not found in creosote preservatives, the products of creosote preservatives' oxidation-reduction reactions include some of these compounds. For oxidation-reduction reactions, see the following section. In this study, it was seen that certain compounds such as benzene were only reduced under sulfate-enriched environments, while toluene was reduced under a variety of bacteria-enriched environments, not just sulfate.[26] The biodegradation of a creosote preservative in an anaerobic enrichment depends not only on the type of bacteria enriching the environment, but also the compound that has been released from the preservative. In aerobic environments, preservative compounds are limited in the biodegradation process by the presence of free oxygen.[27] In an aerobic environment, free oxygen comes from oxygen saturated sediments, sources of precipitation, and plume edges.[27] The free oxygen allows for the compounds to be oxidized and decomposed into new intermediate compounds.[27] Studies have shown that when BTEX and PAH compounds were placed in aerobic environments, the oxidation of the ring structures caused cleavage in the aromatic ring and allowed for other functional groups to attach.[27] When an aromatic hydrocarbon was introduced to the molecular oxygen in experimental conditions, a dihydrodiol intermediate was formed, and then oxidation occurred transforming the aromatic into a catechol compound.[27] Catechol allows for cleavage of the aromatic ring to occur, where functional groups can then add in an ortho- or meta- position.[27]
Oxidation-Reduction
Even though many studies conduct testing under experimental or enriched conditions, oxidation-reduction reactions are naturally occurring and allow for chemicals to go through processes such as biodegradation, outlined above. Oxidation is defined as the loss of an electron to another species, while reduction is the gaining of an electron from another species. As compounds go through oxidation and reduction in sediments, the preservative compounds are altered to form new chemicals, leading to decomposition. An example of the oxidation of p-cresol and phenol can be seen in the figures below:
p-Cresol
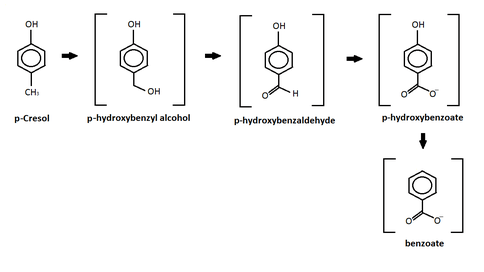
This reaction shows the oxidation of p-cresol in a sulfate-enriched environment.[28] P-cresol was seen to be the easiest to degradete through the sulfate-enriched environment, while m-cresol and o-cresol where inhibited.[28] In the chart above, p-cresol was oxidized under an anaerobic sulfate reducing condition and formed four different intermediates.[28] After the formation of the intermediates, the study reported further degradation of the intermediates leading to the production of carbon dioxide and methane.[28] The p-hydroxylbenzyl alcohol, p-hydroxylbenzaldehye, p-hyrdoxylbenzoate, and benzoate intermediates all are produced from this oxidation and released into the sediments.[28] Similar results were also produced by different studies using other forms of oxidation such as: iron-reducing organisms,[29] Copper/Manganese Oxide catalyst,[30] and nitrate- reducing conditions.[31]
Phenol
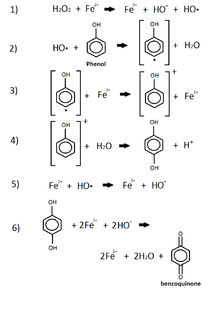
This reaction shows the oxidation of phenol by iron and peroxide.[32] This combination of iron, which comes from iron oxide in the sediment, and the peroxide, commonly released by animals and plants into the enviroment, is known as the Fenton Reagent. [32] This reagent is used to oxidize phenol groups by the use of a radical hydroxide group produced from the peroxide in the p-benzoquinone.[32] This product of phenol's oxidation is now leached into the environment while other products include iron(II) and water. P-benzoquinone is listed as being a very toxic, acute environmental hazard.[33]
Environmental hazards
Sediment
In aquatic sediments, a number of reactions can transform the chemicals released by the creosote preservatives into more dangerous chemicals. Most creosote preservative compounds have hazards associated with them before they are transformed. Cresol (m-,p-, and o-), phenol, guaiacol, and xylenol (1,3,4- and 1,3,5-) all are acute aquatic hazards prior to going through chemical reactions with the sediments. Alkylation reactions allows for the compounds to transition into more toxic compounds with the addition of R-groups to the major compounds found in creosote preservatives. Compounds formed through alkylation include: 3,4-dimethylphenol, 2,3-dimethylphenol, and 2,5-dimethylphenol, which are all listed as acute environmental hazards.[13] Biodegradation controls the rate at which the sediment holds the chemicals, and the amount of reactions that are able to take place. The biodegradation process can take place under many different conditions, and vary depending on the compounds that are released. Oxidation-reduction reactions allow for the compounds to be broken down into new forms of more toxic molecules. Studies have shown oxidation-reduction reactions of creosote preservative compounds included compounds that are listed as environmental hazards, such as p-benzoquinone in the oxidation of phenol.[32] Not only are the initial compounds in creosote hazardous to the environment, but the byproducts of the chemical reactions are environmental hazardous as well.
Other
From the contamination of the sediment, more of the ecosystem is effected. Organisms in the sediment are now exposed to the new chemicals.[34] Organisms are then ingested by fish and other aquatic animals.[34] These animals now contain concentrations of hazardous chemicals which were secreted from the creosote. Other issues with ecosystems include bioaccumulation. Bioaccumulation occurs when high levels of chemicals are passed to aquatic life near the creosote pilings. Mollusks and other smaller crustaceans are at higher risk because they are directly attached to the surface of wood pilings that are filled with creosote preservative. Studies show that mollusks in these environments take on high concentrations of chemical compounds which will then be transferred through the ecosystems food chain. Bioaccumulation contributes to the higher concentrations of chemicals within the organisms in the aquatic ecosystems.
Remedies
Even though creosote preservatives where outlawed by the EPA in 2008, citizens still have a service to the aquatic environment. Creosote treated wood is no long used in the building of structures and piers, but old broken down piers still could contain these creosote preservatives. Many properties contain piers that were built before 2008 with creosote preservatives, and now remain in the water even if they are broken down. One simple remedy for this would be removing the pilings after they are broken down or are no longer in use. On the coast, after storms pass through, debris and wreckage breaks piers that are built on the water. One of the harder remedies is for pilings that have sunk to the bottom of the water and settled on the sediment. These pilings are not visible and are harder to detect. The pilings will then sit on the bottom and leach chemicals out into the sediment and ecosystem. A solution to the problem of hidden pilings could be an analytical method or technique that could be used to track creosote compounds or byproducts in situ (the original place of contamination). If there was a technique that could be used out in the field that could trace higher concentrations of the chemical in the sediment, then hidden pilings could be isolated and removed from the environment. Many methods, such as gas chromatography-mass spectroscopy (GCMS) and high performance liquid chromatography (HPLC), have been used to identify creosote preservatives in the ground water and sediment, but most methods must be taken back to the lab in order to be properly conducted due to the run time and size of the machine.[35] New studies have shown that the use of smaller, more user friendly bio-assays are available to researchers so they can be used in the field for faster identification of chemical compounds.[35] A test that could identify creosote compounds or other toxic byproducts quickly and efficiently in the field would allow reseachers to remove contaminated pilings before further damage can be done.
Related content
References
- ↑ "Public Health Statement for Creosote". Toxic Substance Portal-Creosote. Agency for Toxic Substance and Disease Registry. September 2002. Retrieved October 8, 2016.
- ↑ "Pesticide Fact Sheet". Environmental Protection Agency. National Service Center for Environmental Publiciations. July 11, 1984. Retrieved November 21, 2016.
- 1 2 3 4 "Creosote". Ingredients Used in Pesticide Products. US Environmental Protection Agency. May 26, 2016. Retrieved October 8, 2016.
- 1 2 Choudhary, Gangadhar (September 2002). "Toxicological Profile for Wood Creosote, Coal Tar Creosote, Coal Tar, Coal Tar Pitch, and Coal Tar Pitch Volatiles" (PDF). Toxic Profile. U.S. Department of Health and Human Service. Retrieved October 8, 2016.
- 1 2 3 4 5 6 7 8 9 Freeman, Michael (October 2003). "Past, Present, and Future of the Wood Preservation Industry" (PDF). Feature. Forest and Wildlife Research Center-Mississippi State. Retrieved October 8, 2016.
- 1 2 Zasshi, Yakushigaki (October 7, 2001). "Wood Creosote: A Historical Study and Its Preparation in Combination with Herbal Drugs". PubMed.gov. National Center for Biotechnological Information. Retrieved October 8, 2016.
- ↑ "Overview of Wood Preservative Chemicals". Ingredients Used in Pesticide Products. US Environmental Protection Agency. May 26, 2016. Retrieved October 8, 2016.
- 1 2 Shupe, Todd (June 2008). "Causes and Control of Wood Decay, Degradation and Stain" (PDF). LSU Agricultural Center Research and Extension. Retrieved October 28, 2016.
- 1 2 Smith, Stephen (May 31, 2002). "Environmental Issues Related to the Use of Creosote Wood Preservative". Research Gate. AquAeTer. Retrieved October 28, 2016.
- 1 2 Shupe, Todd (September 27, 2012). "Marine Wood Borers". LSU Agricultural Center Research and Extension. Retrieved October 28, 2016.
- ↑ "Alkylation". Dictionary.com. Dictionary.com. Retrieved October 29, 2016.
- 1 2 Connell, Des (July 14, 2005). "Basic Concepts of Environmental Chemistry, Second Edition". Google Books. Taylor and Francis CRC. Retrieved October 29, 2016.
- 1 2 3 4 5 6 Bolognini, M (July 2002). "Heterogeneous Basic Catalysts as Alternative to Homogeneous Catalysts:Reactivity of Mg/Al mixed Oxides in the Alkylation of m-Cresol with Methanol". Research Gate. Retrieved October 28, 2016.
- ↑ Howard, Phillip (February 28, 1990). "Handbook of Environmental Fate and exposure Data for Organic Chemicals, Volume 2". Google Books. Lewis Publisher. Retrieved October 28, 2016.
- ↑ "2,3-Dimethylphenol". Open Chemistry Database. PubChem. Retrieved October 29, 2016.
- 1 2 Balsama, S (December 14, 1984). "Alkylation of Phenol with Methanol over Zeolites". Science Direct. Applied Catalysis. Retrieved October 29, 2016.
- ↑ "Anisole". Compound Summary for CID 7519. PubChem. Retrieved October 29, 2016.
- 1 2 Clarke, Joan (July 1991). "Assessing Bioaccumulation in Aquatic Organisms Exposed to Contaminated Sediments" (PDF). US Army Corps of Engineers. Retrieved October 29, 2016.
- ↑ "Creosote Release from Cut/broken Piles, Asarco Smelter Site" (PDF). Department of Natural Resources. June 2011. Retrieved October 29, 2016.
- 1 2 3 4 Elder, J.F. (1988). "Accumulation and bioconcentration of polycyclic aromatic hydrocarbons in a nearshore estuarine environment near a Pensacola (Florida) creosote contamination site". USGS Publication Warehouse. United States Geological Survey. Retrieved October 29, 2016.
- 1 2 3 4 5 6 Neff, J.M. (April 16, 2002). "Bioaccumulation in Marine Organisms: Effect of Contamination from Oil Well Produced Water". Google Books. ElSevier. Retrieved October 29, 2016.
- ↑ "Ocean Acidification". Pristine Seas. National Geographic. 2015. Retrieved October 29, 2016.
- 1 2 "Reregistration Eligibility Decision for Creosote (Case 0139)" (PDF). Environmental Protection Agency. September 25, 2008. Retrieved October 29, 2016.
- 1 2 3 Han, Fengxiang (September 2016). "Biodegradation". Journal of Bioremediation and Biodegradation Open Access. Journal of Bioremediation and Biodegradation. Retrieved October 30, 2016.
- 1 2 3 Ramanand, K (June 1991). "Anaerobic degradation of m-cresol in anoxic aquifer slurries: carboxylation reactions in a sulfate-reducing bacterial enrichment". Applied and Environmental Microbiology. US National Library of Medicine National Institute of Health. Retrieved November 2, 2016.
- 1 2 3 Phelps, C (February 1999). "Anaerobic biodegradation of BTEX and gasoline in various aquatic sediments". PubMed.gov. US National Library of Medicine National Institute of Health. Retrieved November 2, 2016.
- 1 2 3 4 5 6 Aronson, Dallas (January 27, 1999). "Aerobic Biodegradation of Organic Chemicals in Environment Media: A Summary of Field and Laboratory Studies" (PDF). Environment Science Center Syracuse Research Corporation. Retrieved November 2, 2016.
- 1 2 3 4 5 Smolenski, Walter (April 1987). "Biodegradation of Cresol Isomer in Anoxic Aquifier" (PDF). Applied and Environmental Microbiology. Retrieved November 3, 2016.
- ↑ Lovley, Derek (June 1990). "Anaerobic Oxidation of Toluene, Phenol, and p-Cresol by the Dissimilatory Iron-Reducing Organism, GS-15". Applied and Environmental Microbiology. American Society for Microbiology. Retrieved November 3, 2016.
- ↑ Wang, Feng (May 2004). "Oxidation of p-Cresol to p-Hydroxybenzaldehyde with Molecular Oxygen in the Presence of CuMn-Oxide Heterogeneous Catalyst". Wiley Online Library. Advanced Synthesis and Catalysis. Retrieved November 3, 2016.
- ↑ Bossert, I (November 1986). "Anaerobic oxidation of p-cresol by a denitrifying bacterium". PubMed.gov. Us National Library of Medicine National Institute of Health. Retrieved November 3, 2016.
- 1 2 3 4 Zazo, J (October 26, 2005). "Chemical Pathway and Kinetics of Phenol Oxidation by Fenton's Reagent". ACS Publications. Environmental Science and Technology. Retrieved November 3, 2016.
- ↑ "p-benzoquinone". Compound Summary for CID 4650. PubChem Open Chemistry Database. Retrieved November 3, 2016.
- 1 2 "Aquatic Food Webs". Marine Life Education Resource. National Oceanic and Atmospheric Administration. Retrieved November 6, 2016.
- 1 2 Hartnik, Thomas (January 2007). "Bioassay-directed identification of toxic organic compounds in creosote-contaminated groundwater". Science Direct. Chemosphere. Retrieved November 8, 2016.